Photovoltaic tweezers based on LiNbO3 crystal and non-diffracting Bessel beams: Trapping and manipulation of micro/nano-objects
Research Background:
The particles manipulation techniques are important in many fields of research, from integrated optics and photonic devices to micro- and nanoelectronics and biotechnology. Laser assisted methods for trapping and manipulation of micro- and nanoparticles have experienced impressive development last decades.
Optical tweezers are well established tools for trapping of particles and/or atoms and molecules by focused laser beams and organizing them in the ordered arrangements. Conventional optical tweezers rely on the laser field gradient near the focus of optical beam which gives rise to the trapping force toward the focus. Optical tweezers are used to manipulate single particle with high accuracy, but because of high intensity required parallel manipulation of many particles is not feasible.
Neutral particles also can be manipulated electro-kinetically via dielectrophoretic (DEP) forces by static electric fields provided by electrodes. This method allows the increase of the number of manipulating particles, but due to the static electrodes the method lacks flexibility. Moreover, to provide the large values of electric fields of the order of 106 V/m a micrometric lithography technique is required for design and fabrication of microelectrodes.
Novel approach is the combination of optical and electro-kinetic methods using photorefractive crystals. The essence of the effect relates to the very strong space charge electric fields (~107 V/m) generated in the volume and proximity of the crystal by spatially inhomogeneous illumination of photorefractive crystals and associated with bulk photovoltaic effect. This approach has shown a great promise for trapping and manipulation of large number of micro- and nanoparticles on the surface of the crystal. Such trapping devices were called photovoltaic tweezers. Photovoltaic tweezers allow the manipulation in parallel of a very large number of particles and prepare functional structures. In photorefractive crystals the photovoltaic electric fields remain very long time (up to the few months, depending on dark conductivity and doping level) providing the stability of structures. Strong photovoltaic fields allow also the manipulation of the massive objects which is promising for biotechnology.
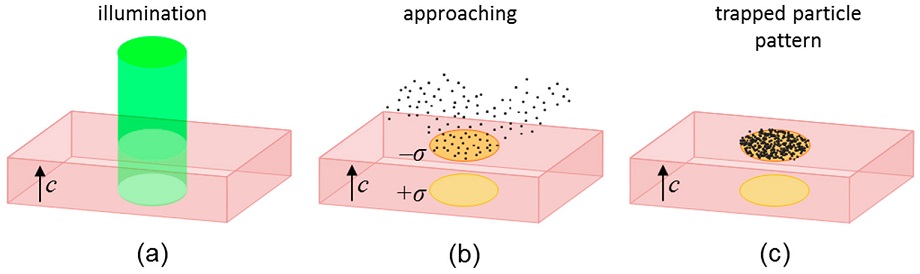
Research Progress:
The novel approach of photovoltaic tweezers is based on the optical holography technique. The illumination of photorefractive crystals by spatially modulated laser light redistributes photo-charges, excited by photo-ionization and builds up internal space charge electric fields ESC via photovoltaic effect. Space charge fields induced in photorefractive crystals by inhomogeneous illumination modulates the refractive index by electro-optic effect thus creating the refractive structures inside the crystal. The geometry of refractive structure replicates the laser light illumination pattern. However, the light-induced space charge (photovoltaic) field not only affects the refractive index inside the crystal but also serve as a source of periodically distributed electric fields near its surface. The generated electric fields can act on micro- or nano-objects when they are close enough to the crystal surface. The photovoltaic evanescent electric fields E induce electrical forces on either the particles that have electrical charge q (electrophoretic forces FEP = qE) or the neutral particles that become polarized by electric field (dielectrophoretic forces): FDEP ~ P×? E, where E is the photovoltaic field, P is the polarization of medium, ? denotes the gradient.
The aim of the Project is the design and fabrication of a chip-scale photovoltaic tweezers based on the photorefractive lithium niobate (LN) crystal and non-diffracting Bessel beam technique for trapping and manipulation of micro- and nanoparticles.
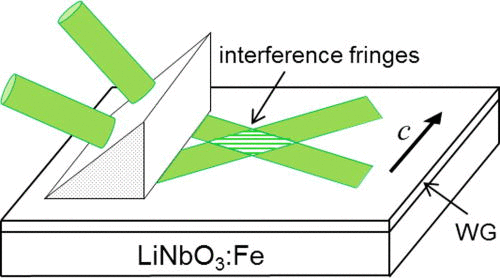
Among different methods of holographic recording of photonic lattices the non-diffracting optical beams [8] are of special interest because have a feature of conserving their transverse intensity distribution during propagation in free space. This feature makes diffraction-free beams very promising for formation of high contrast volume photonic structures. Bessel beam with concentric rings structure is a fundamental example of non-diffracting optical beams.
The novelty and originality of the project is the use of cw non-diffracting optical Bessel beams for formation of specific high contrast 2D space charge electric fields (~107 V/m) in the volume and proximity of surface of the photorefractive crystal thus providing 2D trapping and manipulation of micro/nano particles on the crystal surface. As a basic crystalline material for photovoltaic trapping the Fe-doped lithium niobate (LN:Fe) crystal will be used because of its excellent photorefractive properties. The first results of trapping of dielectric microparticles and Ag nanoparticles on LN:Fe crystal by optical Bessel beam induced space-charge field were reported by our group in.
The LN:Fe crystal with Bessel beam induced photovoltaic fields enabling particle trapping and manipulation on the crystal surface can be considered as a chip-scale photovoltaic tweezers. Such lab-on-a-chip device will be especially promising for observation of trapping, manipulations of biological objects in suspensions. The LiNbO3:Fe based photovoltaic tweezers are good platform for biomedical studies [16].
Our activity as a whole will develop in the following main directions: (i) growth of doped photorefractive lithium niobate crystals which meet special requirements, (ii) generation of strong and high-contrast space charge fields in doped LN crystals by Bessel beam technique for elaboration of photovoltaic tweezers, (iii) trapping of micro- and nano-size dielectric and metal particles and their suspensions on lithium niobate substrate with Bessel beam induced strong space charge field near the crystal surface. (iv) Formation of 2D concentric ring microfluidic channels on the surface of LN:Fe crystal by Bessel beam technique. (v) Extension of the photovoltaic trapping method to biological objects (e.g. leukocytes, erythrocytes, DNA molecules etc).
Demand for Collaboration:
The following modes of cooperation can be considered:
-joint research activities
-exchange of researchers
-joint participation in conferences and seminars
-exchange of ideas, information and samples
-preparation of joint scientific and innovation projects
-joint publications
We can consider also some educational aspects of cooperation:
-Visits of professors for short courses in the fields of photonics, laser physics and nonlinear optics
-Joint Master and PhD programs
Benefits:
(i) Main impact of the Project is the development of the novel lab-on-a-chip technology for trapping and manipulation of both single and large number of micro- and nanoparicles. The technology is based on the LN:Fe crystals (10 mm ′10 mm ′2 mm) with recorded Bessel lattice (2-4 mm in diameter and 10-60 mm periodicity of Bessel rings) and photovoltaic field distribution on its surface enabling particle trapping and manipulation .
(ii) Lab-on-a-chip device can be integrated in any type of optical microscope for observation and real time study.
(iii) Complementary expertise of the teams in Photonics, Material Science and Biophysics will be shared within the bilateral collaboration.
Socio-economic Impact
(i) Commercialization of the lab-on-a chip after successful realization of the Project is foreseen.
(ii) Realization of the Project will gain an experienced cadre of young researchers with crucial capabilities, who can stimulate new applications and advances in novel photonic materials, micro/nanoelectronics and biotechnologies.